Cooling off with lasers
Lasers are typically thought of as hot. What if they were able to cool?
Professor Stephen Rand is researching a concept that, to most people, is counter-intuitive. “The basic idea of this project is to use light to cool things,” says Rand. “Most people think lasers always heat, but it depends on how the wavelength of the light is tuned.” Specifically, Rand and his team are studying how to use lasers to cool down solid matter.
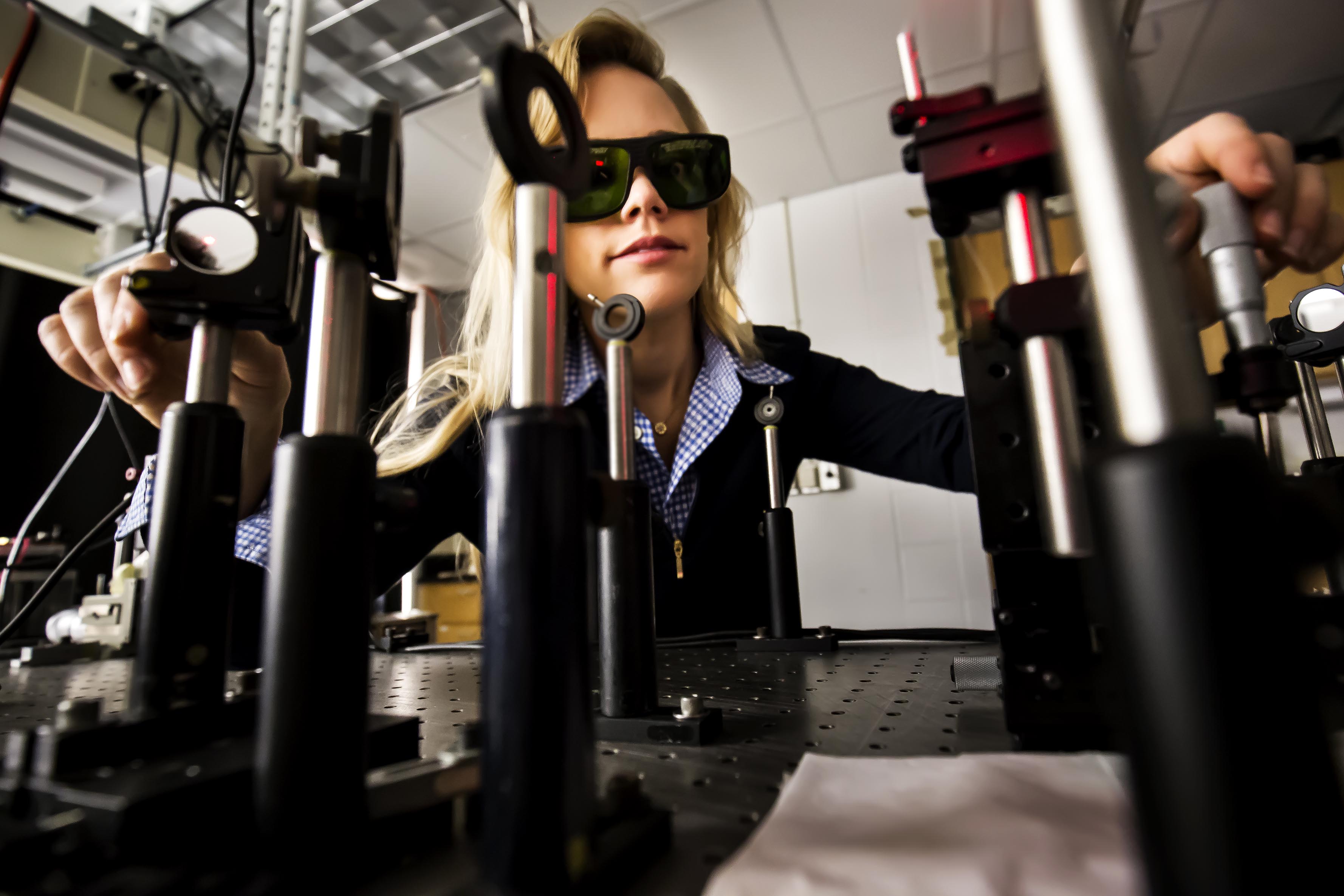
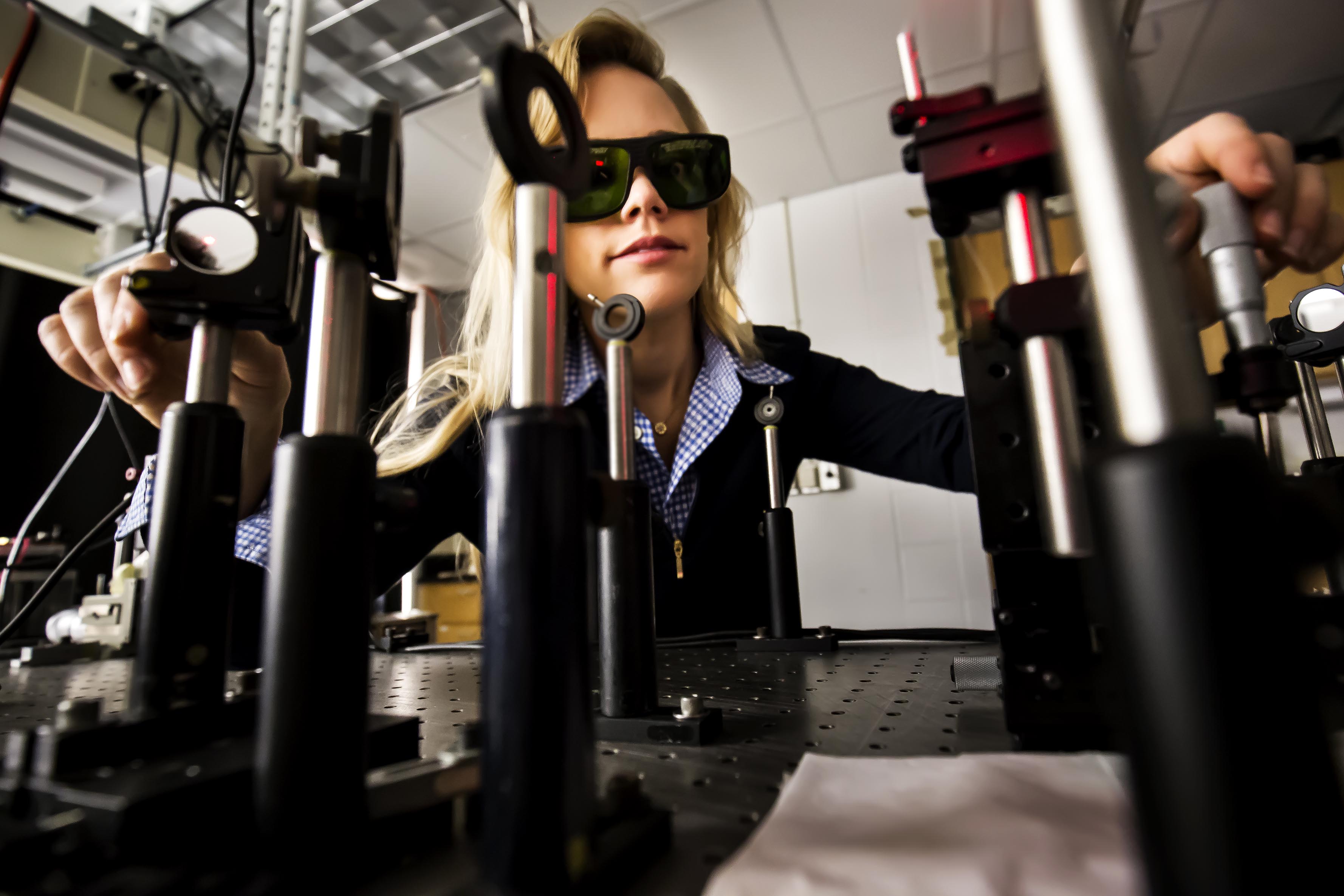
The idea isn’t new: Peter Pringsheim, a German professor of physics, wrote a paper outlining how cooling with light could work within the laws of thermodynamics in 1929. And, Steven Chu, Claude Cohen-Tannoudji, and William Phillips won the 1997 Nobel Prize in Physics for their work in laser cooling of gasses. However, the U-M team’s approach is new, and should be able to achieve an unprecedented cooling of solid matter to open up new applications for lasers and imaging arrays in space.
Lasers typically heat objects by adding energy to a material. This happens because the energy of the light wave increases the motion of target atoms, which increases the material’s temperature.
But, just as a laser can make a material’s atoms move faster and increase temperature, it can also make them move slower. Depending on the composition of the target and the wavelength of the light, a laser can oppose the motion of the target material’s atoms. The photons can push on target atoms in a way to remove energy, and thereby reduce a material’s temperature.
Besides breaking common notions about lasers, there are several applications for the refrigeration of solids with light.
One hope is to create a self-cooling, or radiation-balanced, laser. When attempting to create more powerful lasers, one enemy is heat. As a laser heats up, the gain medium becomes distorted by thermal energy, and this limits the attainable power. With a self-cooling laser, the heat that hinders more powerful lasers would be mitigated, and cumbersome methods of cooling based on circulation of water could be avoided.
Another application is for clearer pictures from space. In space, the quality of imagery with semiconductor arrays is affected by thermal noise. This noise reduces the sensitivity of the cameras. Currently implemented technology can only cool the circuits of these arrays to about 140 K, or -270 ˚F, far from the potential temperature that Rand’s approach could achieve of 1 K.
INFOBAND
Additionally, lasers could offer a low-maintenance and non-contact method of cooling, important for extreme environments and sensitive equipment.
In testing, Mansoor Sheik-Bahae, professor of physics and astronomy at University of New Mexico, and his team achieved laser cooling to 90 K. However, these tests utilize what’s known as anti-Stokes fluorescence, and as the temperature drops, the rate of cooling also decreases. This sets a limit on temperatures to which the anti-Stokes process can cool.
The new technique pioneered by Rand and his team utilizes a Raman process, which should theoretically be able to maintain a constant rate of cooling. The process allows optimizing both the absorption of light by the material and its subsequent fluorescence, which is how the energy and heat is removed. This could bring the temperature to the theoretical limit of 1 K.
Laura Andre, one of Rand’s PhD students, notes one of the challenges they will need to overcome as they move from theory to experimentation. “An interesting issue is how to measure the temperature of sample,” Andre says.
“When the atom decays, it emits a photon, which we call fluorescence,” she continues. “That’s what’s taking away the energy. We need a way to measure temperature that’s not going to interfere with fluorescence, otherwise it will be reflected back and generate more heat.”
Another student in Rand’s research group, Long Cheng, is working with Andre to use the spectrum of emitted light itself to measure sample temperature. Jointly, they recently cooled a crystal by nearly 2 K in the open lab.
The project is funded by the Department of Defense as part of a Multidisciplinary University Research Initiative (MURI). The MURI is led by University of Illinois, and includes Clemson University, Stanford, and U-M. Rand is also Director of the Center for Dynamic Magneto-Optics (DYNAMO).